Recently, Professor Liang Bin, Professor Cheng Jianchun and their team from Institute of Acoustics, Department of Physics made new progress in remote water-to-air eavesdropping, and published a research paper titled “Remote Water-to-Air Eavesdropping with a Phase-Engineered Impedance Matching Metasurface” on Advanced Materials (https://doi.org/10.1002/adma.202301799). Associate Researcher Liu Jingjing from Institute of Acoustics, Department of Physics is the first author, while Professor Liang Bin, Professor Cheng Jianchun, and Professor Andrea Alù from the City University of New York, USA, are the co-corresponding authors of the paper.
The abstract of the paper is as following:
Efficiently receiving underwater sound remotely from air is a long-standing challenge in acoustics hindered by the large impedance mismatch at the water–air interface. Here, a phase-engineered water–air impedance matching metasurface is proposed and experimentally demonstrated for remote and efficient water-to-air eavesdropping. The judiciously designed metasurface with near-unity transmission efficiency, long monitoring distance, and high mechanical stiffness is capable of making the water–air interface acoustically transparent and, at the same time, freewheelingly patterning the transmitted wavefront. This enables efficient control over the effective spatial location of a distant airborne sensor such that it can measure underwater signals with large signal-to-noise ratios as if placed close to the physical underwater source. Such airborne eavesdropping of underwater sound is experimentally demonstrated with a measured sensitivity enhancement of nearly 104 at 8 kHz, far from achievable with the current state-of-the-art methods. Moreover, the opportunities of using the proposed metasurface for cross-media orbital-angular-momentum-multiplexed communication and underwater acoustic window are also demonstrated. This metasurface opens new avenues for communication and sensing in inhomogeneities with totally reflective interfaces, which may be translated to nano-optics and radio frequencies.
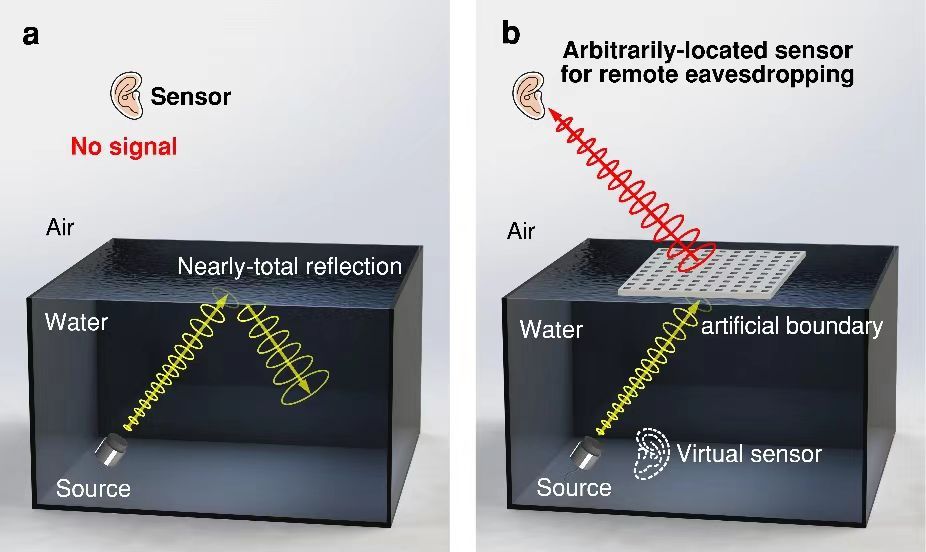
Figure 1 Impedance matching metasurface for remote water-to-air eavesdropping. a) Schematic diagram of airborne monitoring of underwater signals at the water–air interface. b) Proposed principle for remote water-to-air eavesdropping through impedance matching metasurface with subwavelength thickness. By judiciously decorating the interface with a tailored artificial boundary, extreme enhancement in sensitivity can be achieved.
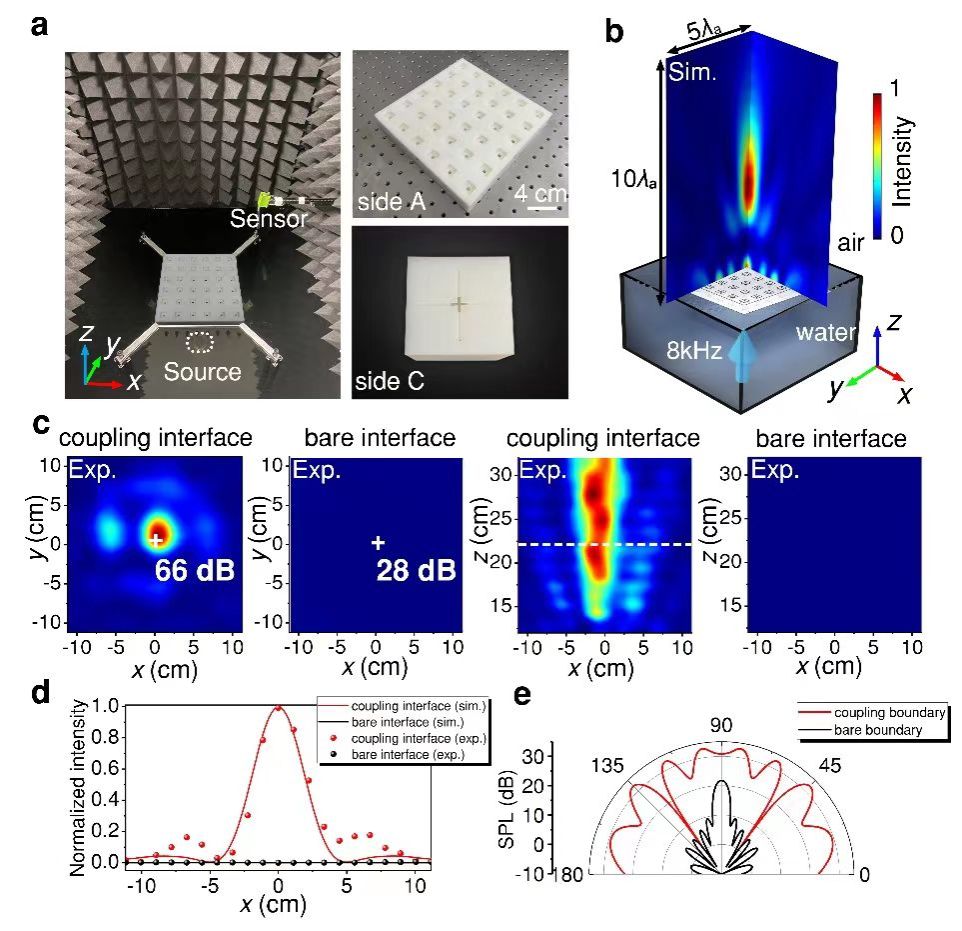
Figure 2. Experimental realization of high-SNR airborne eavesdropping for low-frequency underwater signals. a) Photograph of experimental setup and fabricated sample. The 3D size of the water tank is 0.8 × 0.8 × 0.8 m3. b) Left: Simulated 3D sound intensity distribution of transmitted sound wave in air. Right: Simulated sound pressure and intensity distributions of whole calculating area in the x–z plane. c) Measured sound intensity distributions over 20 × 20 cm2 square regions in the focal plane and in the vertical plane with and without metasurface. d) Comparison between measured and simulated sound intensity profiles on the x-axis (white straight line in (c)). e) Simulated far-field SPL with and without metasurface.
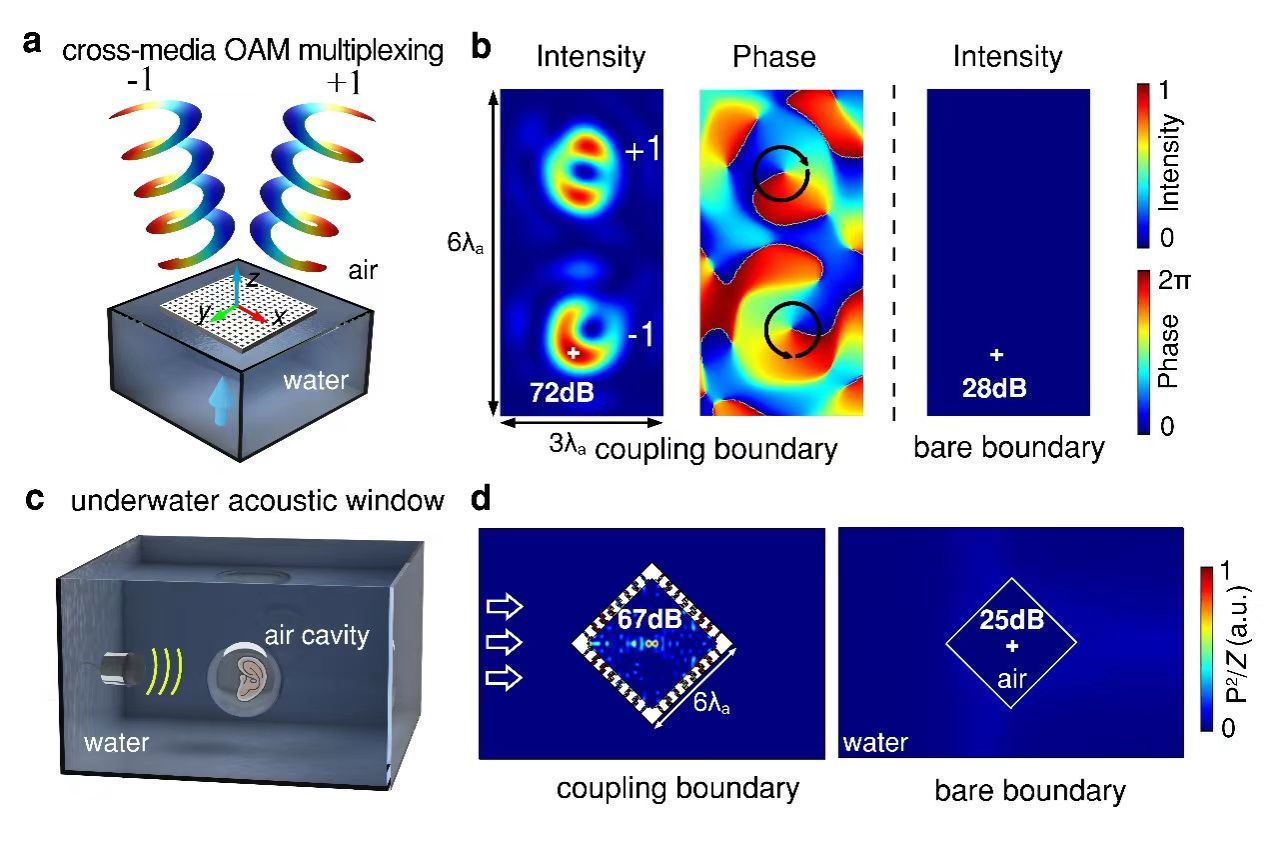
Figure 3. Demonstration of cross-media OAM multiplexing and underwater acoustic window. a) Schematic of cross-media OAM multiplexing (with orders of 1 and −1 as an example). b) Simulated intensity and phase distributions on the detection plane for OAM multiplexing with and without metasurface. c) Schematic of the acoustic window for underwater eavesdropping. d) Simulated 2D intensity distributions for underwater eavesdropping inside a square air cavity with and without metasurface, respectively. The white arrows denote the incident waves in water.
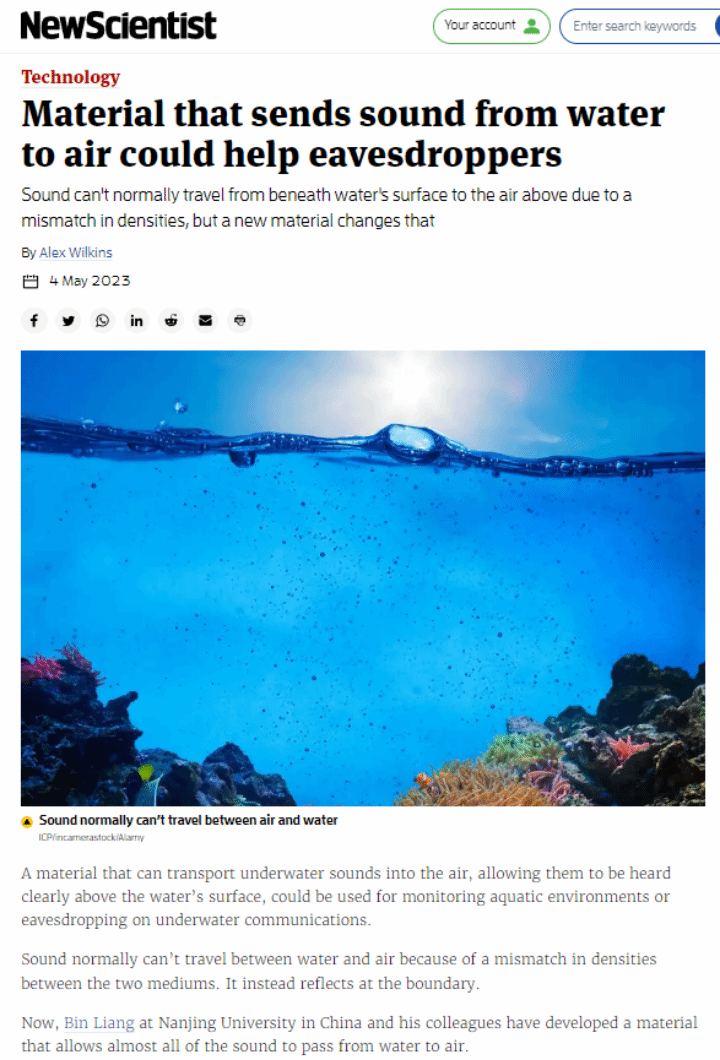
Figure 4. The paper is reported by New Scientist.
Editors: Guo Ankang, Li Jiesheng